There are numerous carbon nanotubes properties and applications which take full advantage of CNTs aspect ratio, mechanical strength, electrical and thermal conductivity. We’ve compiled the list below for you.
Types of Carbon Nanotubes
The types of carbon nanotubes are typically referred to as Single Walled Carbon Nanotubes and Multi Walled Carbon Nanotubes. If you wish to buy carbon nanotubes, please use the drop down products menu or click the links above. There are many variations of both types. They vary by purity, length, and functionality.
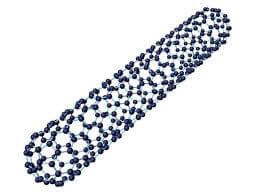
Carbon Nanotubes Properties
Electrical Conductivity
Strength and Elasticity
Thermal Conductivity And Expansion
Electron Emission
Aspect Ratio
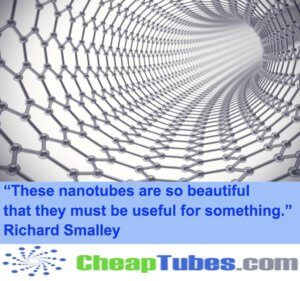
Electrical Conductivity
There has been considerable practical interest in the conductivity of CNTs. CNTs with particular combinations of N and M (structural parameters indicating how much the nanotube is twisted) can be highly conducting, and hence can be said to be metallic. Their conductivity has been shown to be a function of their chirality (degree of twist), as well as their diameter. CNTs can be either metallic or semi-conducting in their electrical behavior.
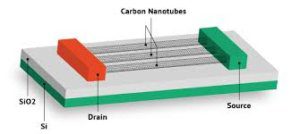
Conductivity in MWNTs is quite complex. Some types of “armchair”-structured CNTs appear to conduct better than other metallic CNTs. Furthermore, interwall reactions within MWNTs have been found to redistribute the current over individual tubes non-uniformly. However, there is no change in current across different parts of metallic single-walled CNTs. However, the behavior of ropes of semi-conducting SWNTs is different, in that the transport current changes abruptly at various positions on the CNTs.
The conductivity and resistivity of ropes of SWNTs has been measured by placing electrodes at different parts of the CNTs. The resistivity of the SWNT ropes was in the order of 10–4 ohm-cm at 27°C. This means that SWNT ropes are the most conductive carbon fibers known. The current density that was possible to achieve was 107 A/cm2, however in theory the SWNT ropes should be able to sustain much higher stable current densities, as high as 1013 A/cm2.
It has been reported that individual SWNTs contain defects. Fortuitously, these defects allow the SWNTs to act as transistors. Likewise, joining CNTs together may form transistor-like devices. A nanotube with a natural junction (where a straight metallic section is joined to a chiral semiconducting section) behaves as a rectifying diode – that is, a half-transistor in a single molecule. It has also recently been reported that SWNTs can route electrical signals at high speeds (up to 10 GHz) when used as interconnects on semi-conducting devices.
Strength And Elasticity
The carbon atoms of a single (graphene) sheet of graphite form a planar honeycomb lattice, in which each atom is connected via a strong chemical bond to three neighboring atoms. Because of these strong bonds, the basal-plane elastic modulus of graphite is one of the largest of any known material.
For this reason, CNTs are expected to be the ultimate high-strength fibers. SWNTs are stiffer than steel, and are very resistant to damage from physical forces. Pressing on the tip of a nanotube will cause it to bend, but without damage to the tip. When the force is removed, the tip returns to its original state. This property makes CNTs very useful as probe tips for very high-resolution scanning probe microscopy.
Quantifying these effects has been rather difficult, and an exact numerical value has not been agreed upon. Using an atomic force microscope (AFM), the unanchored ends of a freestanding nanotube can be pushed out of their equilibrium position and the force required to push the nanotube can be measured. The current Young’s modulus value of SWNTs is about 1 TeraPascal, but this value has been disputed, and a value as high as 1.8 Tpa has been reported. Other values significantly higher than that have also been reported.
The differences probably arise through different experimental measurement techniques. Others have shown theoretically that the Young’s modulus depends on the size and chirality of the SWNTs, ranging from 1.22 Tpa to 1.26 Tpa. They have calculated a value of 1.09 Tpa for a generic nanotube. However, when working with different MWNTs, others have noted that the modulus measurements of MWNTs using AFM techniques do not strongly depend on the diameter. Instead, they argue that the modulus of the MWNTs correlates to the amount of disorder in the nanotube walls. Not surprisingly, when MWNTs break, the outermost layers break first.
Thermal Conductivity And Expansion
New research from the University of Pennsylvania indicates that CNTs may be the best heat-conducting material man has ever known. Ultra-small SWNTs have even been shown to exhibit superconductivity below 20oK. Research suggests that these exotic strands, already heralded for their unparalleled strength and unique ability to adopt the electrical properties of either semiconductors or perfect metals, may someday also find applications as miniature heat conduits in a host of devices and materials.
The strong in-plane graphitic C-C bonds make them exceptionally strong and stiff against axial strains. The almost zero in-plane thermal expansion but large inter-plane expansion of SWNTs implies strong in-plane coupling and high flexibility against nonaxial strains. Many applications of CNTs, such as in nanoscale molecular electronics, sensing and actuating devices, or as reinforcing additive fibers in functional composite materials, have been proposed.
Reports of several recent experiments on the preparation and mechanical characterization of CNT-polymer composites have also appeared. These measurements suggest modest enhancements in strength characteristics of CNT-embedded matrixes as compared to bare polymer matrixes. Preliminary experiments and simulation studies on the thermal properties of CNTs show very high thermal conductivity. It is expected, therefore, that nanotube reinforcements in polymeric materials may also significantly improve the thermal and thermo-mechanical properties of the composites.
Electron Emission
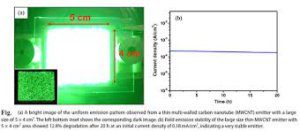
Field emission results from the tunneling of electrons from a metal tip into vacuum, under application of a strong electric field. The small diameter and high aspect ratio of CNTs is very favorable for field emission. Even for moderate voltages, a strong electric field develops at the free end of supported CNTs because of their sharpness.
This was observed by de Heer and co-workers at EPFL in 1995. He also immediately realized that these field emitters must be superior to conventional electron sources and might find their way into all kind of applications, most importantly flat-panel displays. It is remarkable that after only five years Samsung actually realized a very bright color display, which will be shortly commercialized using this technology.
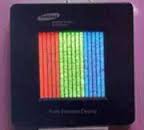
Studying the field emission properties of MWNTs, Bonard and co-workers at EPFL observed that together with electrons light is emitted, as well. This luminescence is induced by the electron field emission, since it is not detected without applied potential. This light emission occurs in the visible part of the spectrum, and can sometimes be seen with the naked eye.
High Aspect Ratio
CNTs represent a very small, high aspect ratio conductive additive for plastics of all types. Their high aspect ratio means that a lower loading (concentration) of CNTs is needed compared to other conductive additives to achieve the same electrical conductivity. This low loading preserves more of the polymer resins’ toughness, especially at low temperatures, as well as maintaining other key performance properties of the matrix resin. CNTs have proven to be an excellent additive to impart electrical conductivity in plastics. Their high aspect ratio (about 1000:1) imparts electrical conductivity at lower loadings, compared to conventional additive materials such as carbon black, chopped carbon fiber, or stainless steel fiber.
Carbon Nanotubes Applications
Their unique composition, geometry, and properties enable numerous potential carbon nanotubes applications. Getting costs down to commercially viable levels has proven challenging but increasing scale is happening.
Energy Storage
Molecular Electronics
Thermal Materials
Structural Materials
Electrical Conductivity
Fabrics And Fibers
Catalyst Supports
Biomedical
Air & Water Filtration
Conductive Plastics
Conductive Adhesives
Ceramics
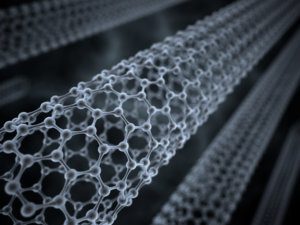
The special nature of carbon combines with the molecular perfection of single-wall CNTs to endow them with exceptional material properties, such as very high electrical and thermal conductivity, strength, stiffness, and toughness.
No other element in the periodic table bonds to itself in an extended network with the strength of the carbon-carbon bond. The delocalized pi-electron donated by each atom is free to move about the entire structure, rather than remain with its donor atom, giving rise to the first known molecule with metallic-type electrical conductivity. Furthermore, the high-frequency carbon-carbon bond vibrations provide an intrinsic thermal conductivity higher than even diamond.
In most materials, however, the actual observed material properties – strength, electrical conductivity, etc. – are degraded very substantially by the occurrence of defects in their structure. For example, high-strength steel typically fails at only about 1% of its theoretical breaking strength. CNTs, however, achieve values very close to their theoretical limits because of their molecular perfection of structure.
This aspect is part of the unique story of CNTs. CNTs are an example of true nanotechnology: they are only about a nanometer in diameter, but are molecules that can be manipulated chemically and physically in very useful ways. They open an incredible range of applications in materials science, electronics, chemical processing, energy management, and many other fields.
Energy Storage
CNTs have the intrinsic characteristics desired in material used as electrodes in batteries and capacitors, two technologies of rapidly increasing importance. CNTs have a tremendously high surface area (~1000 m2/g!!), good electrical conductivity, and very importantly, their linear geometry makes their surface highly accessible to the electrolyte.
Research has shown that CNTs have the highest reversible capacity of any carbon material for use in lithium-ion batteries [B. Gao, Chem. Phys. Lett. 327, 69 (2000)]. In addition, CNTs are outstanding materials for supercapacitor electrodes [R.Z. Ma, et al., Science in China Series E-Technological Sciences 43 178 (2000)] and are now being marketed for this application.
CNTs also have applications in a variety of fuel cell components. They have a number of properties, including high surface area and thermal conductivity, which make them useful as electrode catalyst supports in PEM fuel cells. They may also be used in gas diffusion layers, as well as current collectors, because of their high electrical conductivity.
CNTs’ high strength and toughness-to-weight characteristics may also prove valuable as part of composite components in fuel cells that are deployed in transport applications, where durability is extremely important.
Molecular Electronics
The idea of building electronic circuits out of the essential building blocks of materials – molecules – has seen a revival the past five years, and is a key component of nanotechnology. In any electronic circuit, but particularly as dimensions shrink to the nanoscale, the interconnections between switches and other active devices become increasingly important.
Their geometry, electrical conductivity, and ability to be precisely derived, make CNTs the ideal candidates for the connections in molecular electronics. In addition, they have been demonstrated as switches themselves.
Thermal Materials
CNTs have extraordinary electrical conductivity, heat conductivity, and mechanical properties. They are probably the best electron field-emitter possible. They are polymers of pure carbon and can be reacted and manipulated using the well-known and tremendously rich chemistry of carbon.
This provides opportunity to modify their structure, and to optimize their solubility and dispersion. Very significantly, CNTs are molecularly perfect, which means that they are normally free of property-degrading flaws in the nanotube structure.
Their material properties can therefore approach closely the very high levels intrinsic to them. These extraordinary characteristics give CNTs potential in numerous applications.
The record-setting anisotropic thermal conductivity of CNTs is enabling many applications where heat needs to move from one place to another. Such an application is found in electronics, particularly advanced computing, where uncooled chips now routinely reach over 100oC.
The technology for creating aligned structures and ribbons of CNTs [D.Walters, et al., Chem. Phys. Lett. 338, 14 (2001)] is a step toward realizing incredibly efficient heat conduits. In addition, composites with CNTs have been shown to dramatically increase their bulk thermal conductivity, even at very small loadings.
Structural Materials
The superior properties of CNTs are not limited to electrical and thermal conductivities, but also include mechanical properties, such as stiffness, toughness, and strength. These properties lead to a wealth of applications exploiting them, including advanced composites requiring high values of one or more of these properties.
Electrical Emitters
CNTs are the best known field emitters of any material. This is understandable, given their high electrical conductivity, and the incredible sharpness of their tip (because the smaller the tip’s radius of curvature, the more concentrated will be an electric field, leading to increased field emission; this is the same reason lightning rods are sharp).
The sharpness of the tip also means that they emit at especially low voltage, an important fact for building low-power electrical devices that utilize this feature. CNTs can carry an astonishingly high current density, possibly as high as 1013 A/cm2. Furthermore, the current is extremely stable.
An immediate application of this behavior receiving considerable interest is in field-emission flat-panel displays. Instead of a single electron gun, as in a traditional cathode ray tube display, in CNT-based displays there is a separate electron gun (or even many of them) for each individual pixel in the display.
Their high current density, low turn-on and operating voltages, and steady, long-lived behavior make CNTs very attractive field emitters in this application. Other applications utilizing the field-emission characteristics of CNTs include general types of low-voltage cold-cathode lighting sources, lightning arrestors, and electron microscope sources. [B.Q. Wei, et al, Appl. Phys. Lett. 79 1172 (2001)].
Fabrics And Fibers
Fibers spun of pure CNTs have recently been demonstrated [R.H. Baughman, Science 290, 1310 (2000)] and are undergoing rapid development, along with CNT composite fibers. Such super strong fibers will have many applications including body and vehicle armor, transmission line cables, woven fabrics and textiles. CNTs are also being used to make textiles stain resistant.
Catalyst Supports
CNTs intrinsically have an enormously high surface area; in fact, for SWNTs every atom is not just on a one surface – each atom is on two surfaces, the inside and outside of the nanotube! Combined with the ability to attach essentially any chemical species to their sidewalls (functionalization) provides an opportunity for unique catalyst supports. Their electrical conductivity may also be exploited in the search for new catalysts and catalytic behavior.
Biomedical
The exploration of CNTs in biomedical applications is just underway, but has significant potential. Since a large part of the human body consists of carbon, it is generally though of as a very biocompatible material.
Cells have been shown to grow on CNTs, so they appear to have no toxic effect. The cells also do not adhere to the CNTs, potentially giving rise to applications such as coatings for prosthetics, as well as anti-fouling coatings for ships.
The ability to functionalize (chemically modify) the sidewalls of CNTs also leads to biomedical applications such as vascular stents, and neuron growth and regeneration. It has also been shown that a single strand of DNA can be bonded to a nanotube, which can then be successfully inserted into a cell.
CNTs Air And Water Filtration
Many researchers and corporations have already developed CNT based air and water filtration devices. It has been reported that these filters can not only block the smallest particles but also kill most bacteria. This is another area where CNTs have already been commercialized and products are on the market now.
Conductive Plastics
Much of the history of plastics over the last half-century has involved their use as a replacement for metals. For structural applications, plastics have made tremendous headway, but not where electrical conductivity is required, because plastics are very good electrical insulators.
This deficiency is overcome by loading plastics up with conductive fillers, such as carbon black and larger graphite fibers (the ones used to make golf clubs and tennis rackets). The loading required to provide the necessary conductivity using conventional fillers is typically high, however, resulting in heavy parts, and more importantly, plastic parts whose structural properties are highly degraded.
It’s well-established that the higher the aspect ratio of filler particles, the lower the loading required needed to achieve a given level of conductivity. CNTs are ideal in this sense, since they have the highest aspect ratio of any carbon fiber. In addition, their natural tendency to form ropes provides inherently very long conductive pathways even at ultra-low loadings.
Applications that exploit this behavior of CNTs include EMI/RFI shielding composites; coatings for enclosures, gaskets, and other uses; electrostatic dissipation (ESD); and antistatic materials and (even transparent!) conductive coatings; and radar-absorbing materials for low-observable (“stealth”) applications.
Conductive Adhesives
The same properties that make CNTs attractive as conductive fillers for use in electromagnetic shielding, ESD materials, etc., make them attractive for electronics packaging and interconnection applications, such as adhesives, potting compounds, and coaxial cables and other types of connectors.
CNT Ceramic Materials
A ceramic material reinforced with carbon nanotubes has been made by materials scientists at UC Davis. The new material is far tougher than conventional ceramics, conducts electricity and can both conduct heat and act as a thermal barrier, depending on the orientation of the nanotubes.
Ceramic materials are very hard and resistant to heat and chemical attack, making them useful for applications such as coating turbine blades, but they are also very brittle. The researchers mixed powdered alumina (aluminum oxide) with 5 to 10 percent carbon nanotubes and a further 5 percent finely milled niobium. The researchers treated the mixture with an electrical pulse in a process called spark-plasma sintering. This process consolidates ceramic powders more quickly and at lower temperatures than conventional processes.
The new material has up to five times the fracture toughness — resistance to cracking under stress — of conventional alumina. The material shows electrical conductivity seven times that of previous ceramics made with nanotubes. It also has interesting thermal properties, conducting heat in one direction, along the alignment of the nanotubes, but reflecting heat at right angles to the nanotubes, making it an attractive material for thermal barrier coatings
Other Carbon Nanotubes Applications
There are a wealth of other potential applications for CNTs, such as solar collection; nanoporous filters; catalyst supports; and coatings of all sorts. There are almost certainly many unanticipated applications for this remarkable material that will come to light in the years ahead, and which may prove to be the most important and valuable ones of all. Many researchers are looking into conductive and or water proof paper made with CNTs. CNTs have also been shown to absorb Infrared light and may have applications in the I/R Optics Industry.
References:
-
- “Nanotechnology: Basic Science and Emerging Technologies”, M. Wilson et al, Chapman and Hall (2002) ISBN 1-58488-339-1
-
- “Carbon Nanotubes and Related Structures : New Materials for the Twenty-first Century”, P. F. Harris, Cambridge University Press (1999) ISBN 0-521-55446-2
-
- “Physical Properties of Carbon Nanotubes”, R. Saito et al, Imperial College Press (1998) ISBN 1-86094-093-5
-
- Wondrous World of Carbon Nanotubes (Internet Reference), M. J. M. Daenen et al.
-
- Carbon Nanotube Applications (Internet Reference) www.azonano.com/details.asp?ArticleID=980
-
- “The Science of Fullerenes and Carbon Nanotubes : Their Properties and Applications”, M. S. Dresselhaus et al, Academic Press (1996) ISBN 0-12221-820-5
-
- “Carbon Nanotubes – Preparation and Properties”, T. W. Ebbesen ed., CRC Press (1996) ISBN 0-84939-602-6
-
- “Carbon Nanotubes: Synthesis, Structure, Properties, and Applications”, M. S. Dresselhaus et al eds., Springer-Verlag (2000) ISBN 3-54041-086-4
-
- “Carbon Nanotubes”, T. W. Ebbesen, Ann. Rev. Mater. Sci. 24, 235 (1994); Physics Today 381, 678 (1996)
-
- “Fullerene Nanotubes: C1,000,000 and Beyond”, B. I Yakobson and R. E. Smalley, American Scientist 84(4), 324 (1997)
-
- “Nanotubes from Carbon”, P. M. Ajayan, Chem. Rev. 99, 1787 (1999)
-
- “Carbon Nanotubes : Basic Concepts and Physical Properties”, S. Reich et al, Wiley-VCH (2004) ISBN 3-52740-386-8
- “Physical Properties of Carbon Nanotubes” , R. Saito, World Scientific Publishing (1998) ISBN 1-86094-223-7
- “Carbon Nanotubes: Science and Applications”, M. Meyyappan ed., CRC Press (2004) ISBN 0-84932-111-5
- “Single-shell carbon nanotubes of 1-nm diameter”, S. Iijima and T. Ichihashi, Nature 363 603 (1993)
-
- “Large-scale synthesis of carbon nanotubes”, T. W. Ebbesen and P. M. Ajayan, Nature 358 220 (1992)
-
- Carbon Nanotubes. Noppi Widjaja. Department of Physics, University of Tennessee, Knoxville, TN 37996. Abstract. The field of research in carbon Nanotubes.
-
- [B.Q. Wei, et al, Appl. Phys. Lett. 79 1172 (2001)].
- [B. Gao, Chem. Phys. Lett. 327, 69 (2000)]
- [R.Z. Ma, et al., Science in China Series E-Technological Sciences 43 178 (2000)]
- [D.Walters, et al., Chem. Phys. Lett. 338, 14 (2001)]